Digestion of protein. Features of the process of assimilation of food
All food products are mainly composed of proteins, carbohydrates and lipids. In the process of digestion in the gastrointestinal tract of mammals, the three main components of food: carbohydrates, fats and proteins undergo enzymatic hydrolysis, breaking up into the constituent building blocks from which they are formed. This process is necessary for the disposal of food products, since the cells lining the intestine are able to suck only relatively small molecules into the bloodstream. Assimilation of polysaccharides and even disaccharides becomes possible only after their complete hydrolysis by digestive enzymes to monosaccharides. Similarly, proteins and lipids must also be hydrolyzed to the blocks from which they are built.
The process of digestion begins with the oral cavity and the stomach, whereas the final stages of digestion of all the main components of food and absorption into the blood of the constituent structural units occur in the small intestine. The anatomically small intestine is well adapted to perform this function, since it has a very large surface area through which absorption occurs. The small intestine is characterized not only by a large length of ≈ 4.5 m), but also by the presence on its inner surface of a multitude of folds with a large number of finger-like projections called fibers. Each villus is covered with epithelial cells carrying numerous microvilli. The villi create a huge surface through which digestion products are quickly transported into epithelial cells, and from them into the capillaries of the circulatory system and lymphatic vessels located in the intestinal wall. The surface area of the human small intestine is ≈ 180 m 2, i.e. just a little less than a playground of a tennis court.
The microvilli contain bundles of actin microfilaments connected in the bases of the microvilli with a network of myosin filaments. This system of filaments provides wavelike oscillations of microvilli, due to which local mixing and better absorption of digested nutrients occur.
Protein uptake
Food proteins are digested by enzymes in the gastrointestinal tract to their constituent amino acids (Fig. 1.1). Proteins entering the stomach stimulate the secretion of the hormone gastrin, which in turn causes the secretion of hydrochloric acid by the lining cells of the glands of the gastric mucosa, as well as pepsinogen by the main cells. Gastric juice has a pH from 1.5 to 2.5. Due to this acidity, it acts as an antiseptic, killing most bacteria and other cells. In addition, under conditions of low pH of gastric juice, globular proteins undergo denaturation, their molecules unfold, and as a result, the internal peptide bonds of polypeptide chains become more accessible for enzymatic hydrolysis. Pepsinogen, which is an inactive precursor of the enzyme, or zymogen, is converted in the gastric juice into active pepsin as a result of the enzymatic action of pepsin itself, i.e. by autocatalysis. During this process, 42 are cleaved from the N-terminus of the pepsinogen polypeptide chain.
Activity |
|
(K.F. 3.4.4.1) |
Tyr, Phe, Tgr, Leu, Glu, Gln |
(K.F. 3.4.4.4) |
Attacks peptide bonds formed by: Lys, Arg |
Chymotrypsin (K.F. 3.4.4.5) |
Attacks peptide bonds formed by: Tyr, Phe, Tr p |
Carboxypeptidase (K.F. 3.4.2.1) |
Sequential cleavage of C-terminal residues |
Aminopeptidase (K.F. 3.4.1.1) |
Sequential cleavage of N-terminal residues |
Fig. 1.1. - Protein digestion: 1 - proteolytic enzymes
amino acid residue in the form of a mixture of short peptides. The remaining intact rest of the pepsinogen molecule is an enzymatically active pepsin (KF 3.4.4.1). In the stomach, pepsin hydrolyzes those peptide bonds in proteins that are formed by aromatic amino acids: tyrosine, phenylalanine and tryptophan, as well as a number of others; as a result, a mixture of shorter peptides is formed from long polypeptide chains.
As soon as the acidic contents of the stomach enter the small intestine, secretion of the hormone secretin, which enters the blood, begins in it under the influence of low pH. This hormone in turn stimulates the secretion of bicarbonate from the pancreas into the small intestine, which leads to the neutralization of HC1 in the gastric juice. As a result, the pH rises sharply from 1.5–2.5, to ≈ 7. In the small intestine, the digestion of proteins continues. The entry of amino acids into the duodenum causes the release of the hormone cholecystokinin, which stimulates the secretion of several pancreatic enzymes with a pH optimum of about 7. Three of them: trypsin (K. F. 3.4.4.4), chemotrpsin (K. F. 3.4.4.5) and carboxypeptidase (KF 3.4.2.1) - are produced by exocrine pancreatic cells in the form of enzymatically inactive zymogens: trypsinogen, chymotrypsinogen and procarboxypeptidase, respectively. Due to the synthesis of proteolytic enzymes in the form of inactive precursors, exocrine cells are not destroyed by these enzymes. Once in the small intestine, trypsinogen, under the action of enterokinase, a specialized proteolytic enzyme secreted by cells of the intestinal epithelium, turns into an active form - trypsin. Free trypsin as it is formed also participates in the catalytic conversion of trypsinogen to trypsin. The formation of free trypsin is due to cleavage of the hexapeptide from the N-terminus of the trypsinogen polypeptide chain.
The active center of trypsin consists of three amino acid residues: serine-195 (it is accepted that the numbering of amino acid residues in trypsin corresponds to their positions in the proferment), histidine-57 and aspartic acid-102. The sorption site contains a carboxyl group of aspartic acid-189, which determines the specificity of trypsin to positively charged substrates. The mechanism of catalytic hydrolysis includes the stage of sorption of the substrate, cleavage of the peptide bond with the formation of the acyl enzyme and transfer of the acyl group to the nucleophilic acceptor. Trypsin hydrolyzes peptide bonds formed with the participation of carbonyl groups of lysine and arginine.
Chymotrypsinogen molecule is a single polypeptide chain with several intrachain disulfide bonds. Once in the small intestine, the chymotrypsinogen is converted to chymotrypsin by the action of trypsin, which breaks the long polypeptide chain of chymotrypsinogen in two places, cutting out dipeptides. Three fragments formed from the original chain of chymotrypsinogen, however, are held together by means of cross-disulfide bonds. Chymotrypsin hydrolyzes peptide bonds formed by phenylalanine, tyrosine, and tryptophan residues. Therefore, trypsin and chymotrypsin cleave polypeptides formed in the stomach under the action of pepsin into smaller peptides. This stage of protein digestion proceeds with very high efficiency, since pepsin, trypsin and chymotrypsin exhibit different specificities in the hydrolysis of polypeptide chains with respect to peptide bonds formed by different amino acids.
The degradation of short peptides in the small intestine is carried out by other peptidases. These primarily include carboxypeptidase, a zinc-containing enzyme synthesized in the pancreas as an inactive zymogen of procarboxypeptidase. The active center of carboxypeptidase has the form of a pocket, in the cavity of which the Zn atom is located. The active center also includes residues of glutamic acid, tyrosine, and arginine. The function of the latter in the mechanism of catalysis is the binding of the C-terminal carboxyl group. Carboxypeptidase successively cleaves C-terminal residues from peptides.
The small intestine also secretes aminopeptidase (KF 3.4.1.1), which cleaves N-terminal residues one by one from short peptides.
As a result of the sequential action of these proteolytic enzymes and peptidases, the digestible proteins eventually turn into a mixture of free amino acids, which are then transported through the epithelial cells lining the small intestines. Free amino acids penetrate the capillaries of the villi and are carried by the blood to the liver.
In the human gastrointestinal tract, not all proteins are digested completely. Most animal proteins are almost completely hydrolyzed to amino acids, but a number of fibrillar proteins, such as keratin, are only partially digested. Many proteins of plant foods, in particular proteins of cereal grains, are incompletely split due to the fact that the protein part of the seeds and grains are covered with non-digestible cellulose sheath (husk).
A rare disease of steatorrhea (persistent diarrhea) is known, in which the intestinal enzymes are not able to digest certain water-soluble proteins of the grain, in particular gliadin, which damages the epithelial cells of the intestine. Grain products are excluded from the food of such patients. Another disease associated with abnormal activity of proteolytic enzymes of the digestive tract is acute pancreatitis. In this disease, caused by a violation of the process of excretion of pancreatic juice into the intestine, the precursors of proteolytic enzymes (zymogens) are converted into the corresponding catalytically active forms too early, while still inside the cells of the pancreas.
As a result, these powerful enzymes affect the tissue of the gland itself, causing deep and very painful destruction of the organ, which can be fatal. Normally, zymogens secreted by the pancreas are not activated until they enter the small intestine. The pancreas is protected from self-digestion in another way: a special protein is synthesized in it - a specific trypsin inhibitor. Since free trypsin activates not only trypsinogen and chymotrypsinogen, but also the zymogens of two other digestive enzymes: procarboxypeptidase and proelastase, the trypsin inhibitor successfully prevents the premature formation of free proteolytic enzymes in the cells of the pancreas.
Carbohydrate absorption
In humans, carbohydrates mainly digest polysaccharides: starch and cellulose contained in plant foods. Starch is completely broken down by the enzymes of the gastrointestinal tract to their constituent structural blocks, namely free D -glucose (Fig. 1.2). This process begins in the mouth while chewing food due to the action of the enzyme amylase secreted by the salivary glands. Saliva amylase hydrolyzes many of the α- (1 → 4) -glycoside bonds in starch and glycogen. This forms a mixture consisting of maltose, glucose and oligosaccharides.
Digestion of starch and other digestible polysaccharides to form D -glucose continues and ends in the small intestine, mainly under the action of pancreatic amylase, which is synthesized in the pancreas and enters through the pancreatic duct into the upper small intestine. This section of the small intestine with the highest digestive activity is called the duodenum.
Cellulose in most mammals does not undergo enzymatic hydrolysis and is not used due to the lack of enzymes capable of cleaving the β- (1 → 4) bond between successive residues D - glucose in cellulose. However, undigested cellulose from plant foods creates that mass (sometimes called “fiber” or “roughage”), which contributes to the normal intestinal motility. In ruminants, cellulose is digested, but not directly, but by the action of bacteria in their rumen (stomach). These bacteria hydrolyze cellulose to D - glucose and then fermented D - glucose to lactate, acetate and propionate, which are absorbed and enter the blood. Further, lactate and propionate in the liver of ruminants turn into blood sugar.
Hydrolysis of disaccharides is catalyzed by enzymes located in the outer margin of epithelial cells lining the small intestine. Sucrose, or cane sugar, is hydrolyzed to form D - glucose and D - fructose under the action of sucrase, also called invertase (K.F. 3.2.1.26); lactose is hydrolyzed to D - glucose and D β-galactose under the action of lactase, also called β-galactosidase (K.F. 3.2.1.23); hydrolysis of maltose under the action of maltase produces two molecules D - glucose. Many representatives of Asian and African races in adulthood are characterized by lactose intolerance, due to the disappearance in their small intestine of lactase activity that existed in infancy and childhood. In people with lactose intolerance, this sugar remains in the intestine in an undigested form, and part of it is subjected to digestion under the action of microorganisms. This causes diarrhea and the formation of gas in the intestine.
Fig. 1.2. - Digestion of carbohydrates: 1 and 2 - amylolytic enzymes; 3 - sucrase (K.F. 3.2.1.26); 4 - lactase (K.F. 3.2.1.23)
In the epithelial cells lining the small intestine, D -fructose, D -ractose and D - manna partially transform into D - glucose. A mixture of all these simple hexoses is absorbed by the epithelial cells lining the small intestine and is delivered by blood to the liver.
Fat absorption
The digestion of triacylglycerols (neutral fats) begins in the small intestine, where the zymogen prolipase enters from the pancreas. Here prolipase is converted into active lipase (K. F. 3.1.1.3), which in the presence of bile acids and a special protein called colipase, attaches to triacylglycerol drops and catalyzes the hydrolytic cleavage of one or both extreme fatty acid residues to form a mixture of free fatty acids as their Na + - or K + -sols (soap) and 2-monoacylglycerols. A small amount of triacylglycerols remains at the same time unhydrolyzed (Fig. 1.3).
Fatty acid
Fig. - 1.3. Lipid digestion: 1 - lipase (KF 3.1.1.3), bile acids, Na +
The resulting soaps and undigested acylglycerols are emulsified in the form of small droplets under the action of peristalsis (mixing movements of the intestine), as well as under the influence of salts of bile acids and monoacylglycerols, which are amphipathic compounds and therefore function as detergents. Fatty acids and monoacylglycerols from these droplets are absorbed by intestinal cells, where from them triacylglycerols are mainly synthesized. Further, triacylglycerols do not penetrate into the blood capillaries, but into the small lymphatic vessels of the intestinal villi - lactaeals (otherwise, the milky, or chylous, vessels). Lymph flowing from the small intestines, called chylus (milky sap), after digesting fatty foods resembles milk in appearance due to the abundance of chylomicrons suspended in it - the smallest droplets of emulsified triacylglycerols about 1 micron in diameter. Chylomicrons have a hydrophilic membrane consisting of folipids and a special protein that keeps the chylomicrons in suspension. Chylomicrons pass through the thoracic duct into the subclavian vein. After the consumption of fatty foods, even blood plasma becomes opalescent due to the high concentration of chylomicrons in it, but this opalescence disappears in 1–2 hours, since triacylglycerols are derived from the blood, acting mainly in adipose tissue.
Emulsification and digestion of lipids in the small intestine contribute to bile salts. Human bile salts are mainly sodium glycocholate and sodium taurocholate, both of which are derived from cholic acid, which quantitatively predominates among the four main bile acids present in the human body. Bile salts are powerful emulsifiers; they come from the liver to bile, which is poured into the upper small intestine. After completion of the absorption of fatty acids and monoacylglycerols from emulsified droplets of fat, in the lower part of the small intestine, the bile salts, which contributed to this process, are also reabsorbed. They are returned to the liver and reused. Thus, bile acids constantly circulate between the liver and small intestine.
Bile acids play an extremely important role in the assimilation not only of triacylglycerols, but in general of all fat-soluble components of food. If bile acids are formed or secreted in insufficient quantities, as is the case with a number of diseases, then undigested and unabsorbed fats appear in the feces. At the same time, the absorption of fat-soluble vitamins A, D, E and K worsens and food deficiency of vitamin A may occur.
Split nutrients trapped in the blood are transported to the liver. In hepatic cells - hepatocytes, glucose, amino acids and free fatty acids are included in the body's metabolic processes.
Thus, the process of digestion of food in mammals is carried out in the gastrointestinal tract and is based on enzymatic acid hydrolysis:
· Polysaccharides (starch and cellulose) to di- and monosaccharides,
· Proteins to amino acids
· Lipids to fatty acids, followed by absorption of small intestinal cells into the blood, entering the liver with the bloodstream and inclusion in the metabolic processes of the body.
in the gastrointestinal tract under the action of enzymes of proteases (peptides scrols). The substrate specificity of these enzymes is that each of them with the greatest speed cleaves peptide bonds formed by certain amino acids. Protease, hydrolyzing peptide bonds within a protein molecule, belong to the group of endopeptidases. Enzymes belonging to the exopeptidase group hydrolyze the peptide bond formed by terminal amino acids. Under the action of all proteases of the gastrointestinal tract, food proteins break down into individual amino acids, which then enter tissue cells.
A. Digestion of proteins in the stomach
Gastric juice is a product of several cell types. Lining (parietal) cells of the stomach walls form hydrochloric acid, the main cells secrete pepsinogen. Additional and other epithelial cells of the stomach secrete mucin-containing mucus. Parietal cells also secrete a glycoprotein into the cavity of the stomach, which is called the "internal factor" (Castle factor). This protein binds the "external factor" - vitamin B 12, prevents its destruction and promotes absorption.
1. The formation and role of hydrochloric acid
The main digestive function of the stomach is that it starts digesting protein. A significant role in this process is played by hydrochloric acid. Proteins entering the stomach stimulate secretion histamineand groups of protein hormones - gastrins(see section 11), which, in turn, cause the secretion of HCl and the proenzyme - pepsinogen. HCl is formed in the obladochny cells of the gastric glands in the course of the reactions shown in fig. 9-2.
The source of H + is H 2 CO 3, which is formed in the lining cells of the stomach from CO 2 diffusing from the blood, and H 2 O under the action of the enzyme carbonic anhydrase (carbonate dehydratase):
H 2 O + CO 2 → H 2 CO 3 → HCO 3 - + H +
Dissociation of H 2 CO 3 leads to the formation of bicarbonate, which, with the participation of special proteins, is released into the plasma in exchange for C1 - and H + ions, which enter the lumen of the stomach through active transport catalyzed by membrane H + / K + -ATP-ase. The concentration of protons in the lumen of the stomach increases 10 6 times. C1 ions - enter the lumen of the stomach through the chloride channel.
The concentration of HCl in the gastric juice can reach 0.16 M, due to which the pH value decreases to 1.0-2.0. Eating protein foods is often accompanied by the release of alkaline urine due to the secretion of large amounts of bicarbonate during the formation of HCl.
Fig. 9-2. The secretion of hydrochloric acid in the stomach. 1 - carbonic anhydrase; 2 - H + / K + - ATP-ase; 3 - anion transfer proteins; 4 - chloride channel.
Under the action of HCl, denaturation of food proteins that have not undergone heat treatment occurs, which increases the availability of peptide bonds for proteases. HCl has a bactericidal effect and prevents the ingress of pathogenic bacteria into the intestine. In addition, hydrochloric acid activates pepsinogen and creates an optimum pH for the action of pepsin.
2. Pepsin activation mechanism
Under the action of gastrin in the main cells of the gastric glands, the synthesis and secretion of pepsinogen, an inactive form of pepsin, is stimulated. Pepsinogen is a protein consisting of a single polypeptide chain with a molecular weight of 40 kD. Under the action of HCl, it turns into active pepsin (molecular weight 32.7 kD) with an optimum pH of 1.0-2.5. In the process of activation, as a result of partial proteolysis, 42 amino acid residues are cleaved from the N-terminus of the pepsinogen molecule, which contain almost all the positively charged amino acids present in the pepsinogen. Thus, negatively charged amino acids that are involved in conformational rearrangements of the molecule and the formation of the active center are predominant in active pepsin. The active pepsin molecules formed under the action of HCl quickly activate the remaining pepsinogen molecules (autocatalysis). Pepsin primarily hydrolyzes peptide bonds in proteins formed by aromatic amino acids (phenylalanine, tryptophan, tyrosine) and somewhat slower - formed by leucine and dicarboxylic amino acids. Pepsin is an endopeptidase; therefore, as a result of its action, shorter peptides, but not free amino acids, are formed in the stomach.
3. Age-related digestion of proteins in the stomach
In infants, the enzyme is in the stomach rennin(chymosin), causing milk coagulation. The main milk protein is casein, which is a mixture of several proteins that differ in amino acid composition and electrophoretic mobility. Rennin catalyzes cleavage of the glycopeptide from casein, resulting in the formation of paracasein. Parakazein attaches the ions Ca 2+, forming an insoluble clot, which prevents the rapid release of milk from the stomach. Proteins have time to split under the action of pepsin. There is no rennin in the stomach of adults; their milk is curdled by the action of HCl and pepsin.
Another protease was found in the mucous membrane of the human stomach - gastriksin.All 3 enzymes (pepsin, rennin and gastriksin) are similar in their primary structure, which indicates their origin from the common precursor gene.
4. Violations of digestion of proteins in the stomach
In various gastrointestinal diseases in the stomach, the secretion of HCl and pepsinogen is impaired, while the digestion of proteins is markedly reduced. The most common pathological changes in the acidity of gastric juice. Disturbance of pepsin formation is noted less frequently and detected with more significant lesions of the stomach.
Determination of gastric acidity is used to diagnose various diseases of the stomach (Table. 9-2). Increased aciditygastric juice is usually accompanied by heartburn, diarrhea, and may be a symptom of gastric and duodenal ulcers, as well as hyperacid gastritis. Low acidityit happens with some types of gastritis. The complete absence of HCl and pepsin (gastric ahilia) is observed during atrophic gastritis and is often accompanied by pernicious anemia due to insufficient development of the Castle factor and impaired absorption of vitamin B 12 (see section 3). Anacid(pH of gastric juice\u003e 6.0) indicates a significant loss of the gastric mucosa of the covering cells, secreting hydrochloric acid, which often causes stomach cancer.
The acidity of gastric juice is expressed in titration units (TE) - the amount of 0.1 M NaOH in 1 ml spent on titration of 100 ml of gastric juice according to a certain indicator. In determining the acidity of gastric juice, there are distinguished: total acidity, associated HCl and free HCl.
- The total acidity of gastric juice -the combination of all acid-reactive substances of the gastric juice, is a secret of the stomach, collected within 1 hour.
Table 9-2. Components of gastric juice in normal and pathological conditions
condition | pH | Acidity (TE) | Pepsin | Factor casla | Lactic acid | Blood | ||
common | bound HCl | free HCl | ||||||
Norm | 1,5-2,0 | 40-60 | 20-30 | 20-40 | + | + | - | - |
Hyperacid gastritis | 1,0 | 80 | 40 | + | ± | - | - | |
Hypoacid gastritis | 2,5 | 40 | 20 | ± | ± | ± | - | |
Achilia | 7,0 | 20 | - | - | - | + | - | |
Stomach ulcer | 1,5 | 60 | 40 | + | + | - | + | |
Stomach cancer | 6,0 and\u003e |
40-60 | 20 | + | + | + | + |
Values of total acidity are normally 40-60 TE.
- Associated hydrochloric acid- HCl associated with proteins and their digestion products. Values of bound HCl in healthy people - 20-30 TE.
- Free HCl- hydrochloric acid, not related to the components of the gastric juice. Values of free HCl are normal - 20-40 TE. pH of gastric juice is normal -1,5-2,0.
Lactic acid is normal in the gastric juice is absent. It is formed with a decrease in the content or absence of free hydrochloric acid as a result of the reproduction of lactic acid bacteria or in malignant tumors of the stomach, in cells of which glucose is oxidized anaerobically.
In the diagnosis of diseases of the stomach, in addition to biochemical analyzes, necessarily carry out x-ray and endoscopic examinations, as well as biopsy.
B. Digestion of proteins in the intestines
The gastric contents (chyme) in the process of digestion enters the duodenum. The low pH of the chyme causes the secretion of the secretion of the protein hormone secretin in the blood in the intestine. This hormone in turn stimulates the secretion from the pancreas to the small intestine of pancreatic juice containing HCO 3 -, which leads to the neutralization of HCl in the gastric juice and inhibition of pepsin. As a result, the pH rises sharply from 1.5-2.0 to ∼7.0.
The entry of peptides into the small intestine causes the secretion of another protein hormone, cholecystokinin (see section 11), which stimulates the release of pancreatic enzymes with an optimum pH of 7.5-8.0. Under the action of pancreatic enzymes and intestinal cells, protein digestion is completed.
1. Activation of pancreatic enzymes
In the pancreas, proenzymes of a number of proteases are synthesized: trypsinogen, chymotrypsinogen, proelastase, procarboxypeptidase A and B. In the intestine, they are converted by partial proteolysis into active enzymes trypsin, chymotrypsin, elastase, and carboxepipeptidase A and B.
Activation of trypsinogenoccurs under the action of the intestinal epithelium enzyme enteropeptidase.
This enzyme cleaves the hexapeptide Val- (Asp) 4 -Lyz from the N-terminus of the trypsinogen molecule. A change in the conformation of the remaining part of the polypeptide chain leads to the formation of an active center, and active trypsin is formed. The Val- (Asp) 4 -Liz sequence is inherent in most known trypsinogens of various organisms - from fish to humans.
Formed trypsin activates chymotrypsinogen,from which several active enzymes are obtained (Fig. 9-3). Chymotrypsinogen consists of one polypeptide chain containing 245 amino acid residues and five disulfide bridges. Under the action of trypsin, a peptide bond is cleaved between the 15th and 16th amino acids, resulting in the formation of active π-chymotrypsin. Then, under the action of π-chymotrypsin, the dipeptide ser (14) -arg (15) is cleaved, which leads to the formation of δ-chymotrypsin. Cleavage of the dipeptide trep (147) -arg (148) completes the formation of a stable form of the active enzyme α-chymotrypsin, which consists of three polypeptide chains connected by disulfide bridges.
The remaining pancreatic protease enzymes (proelastase and procarboxypeptidase A and B) are also activated by trypsin by partial proteolysis. The result is the formation of active enzymes - elastase and carboxy-peptidase A and B.
2. The specificity of the action of proteases
Trypsin preferentially hydrolyzes peptide bonds formed by the carboxyl groups of arginine and lysine. Chymotrypsins are most active against peptide bonds formed by the carboxyl groups of aromatic amino acids (Fen, Tyr, Three).
Carboxypeptidases A and B, zinc-containing enzymes, cleave C-terminal amino acid residues. And carboxypeptidase A
Fig. 9-3. Activation of chymotrypsinogen. Chymotrypsinogen molecule consists of 245 amino acid residues and has five disulfide bridges. The diagram shows the areas of the enzyme undergoing proteolysis. a-Chymotrypsin, the active stable form of the enzyme, consists of three polypeptide chains covalently linked by two disulfide bridges and non-covalently due to hydrogen bonds and hydrophobic interactions.
predominantly removes amino acids containing aromatic or hydrophobic radicals, while carboxypeptidase B contains arginine and lysine residues.
The final stage of digestion, hydrolysis of small peptides, occurs under the action of enzymes aminopeptidases and dipeptidases, which are synthesized by the cells of the small intestine in an active form.
- Aminopeptidasesn-terminal amino acids of the peptide chain are successively cleaved. The best known is leucine aminopeptidase - Zn 2+ - or Mn 2+ -containing enzyme, despite the name, which has broad specificity with respect to N-terminal amino acids.
- Dipeptidasethey break down dipeptides into amino acids, but do not act on tripeptides.
As a result of the sequential action of all digestive proteases, most dietary proteins are broken down into free amino acids.
B. Protecting cells against proteases
Pancreatic cells are protected from the action of digestive enzymes by the fact that:
- these enzymes formed as inactive precursorsin pancreatic cells and are activated only after secretion into the intestinal lumen. Thus, the place of synthesis and the site of action of these enzymes are spatially separated.
- in the cells of the pancreas is present trypsin inhibitor protein,forming a strong complex with the active form of the enzyme (in the case of premature activation).
In the cavity of the stomach and intestinal proteases are not in contact with cell proteins, because the mucous membrane is covered with a layer of mucus, and each cell contains on the outer surface of the plasma membrane polysaccharides that are not cleaved by proteases and thereby protect the cell from their action.
The destruction of cellular proteins by proteases occurs in gastric ulcer or duodenal ulcer. However, the initial mechanisms of ulcers are still poorly understood.
G. Transport of amino acids into cells
Amino acids formed during the digestion of proteins are rapidly absorbed in the intestine. Their transport is carried out in two ways: through the portal system of the liver, leading directly to the liver, and through lymphatic vessels, which communicate with blood through the thoracic lymphatic duct. The maximum concentration of amino acids in the blood is reached within 30-50 minutes after ingestion of protein foods (carbohydrates and fats slow down the absorption of amino acids). The absorption of L-amino acids (but not D-isomers) is an active process that requires energy. Amino acids are transferred through the intestinal wall from the mucous membrane of its surface into the blood (Fig. 9-4). The transfer through the brush border is carried out by a number of carriers, many of which operate with the participation of Na + -dependent mechanisms of the symport, like glucose transfer (see section 7).
The different rate of penetration of amino acids through the cell membranes indicates the presence of transport systems that ensure the transfer of amino acids both through the external plasma membrane and through the intracellular membranes. Currently, at least five specific transport systems are known, each of which operates
Fig. 9-4. The mechanism of absorption of amino acids in the intestines. L-amino acid enters the enterocyte by symport with Na + ion. Further, a specific translocase transports an amino acid through the membrane into the blood. The exchange of sodium ions between cells is carried out by primary active transport using Na +, K + -ATP-ases.
for the transfer of a certain group of amino acids similar in structure:
- neutral, with a short side chain (alanine, series, threonine);
- neutral, with a long or branched side chain (valine, leucine, isoleucine);
- with cationic radicals (lysine, arginine);
- with anionic radicals (glutamic and aspartic acid);
- imino acids (proline, hydroxyproline).
Moreover, the number of Na + -dependent are carriers of amino acids in the first and fifth groups, as well as a carrier of methionine. Independent of Na + carriers are specific for some neutral amino acids (phenylalanine, leucine) and amino acids with cationic radicals (lysine).
Amino acids compete with each other for specific binding sites. For example, the absorption of leucine (if its concentration is high enough) reduces the absorption of isoleucine and valine.
One of the specific transport systems for some neutral amino acids functions in the intestines, kidneys and, apparently, the brain. It is called the γ-glutamyl cycle (Fig. 9-5).
In this system, 6 enzymes are involved, one of which is located in the cell membrane, and the rest - in the cytosol. Key role in amino acid transport is played by the membrane-bound enzyme γ - glutamyltransferase.This enzyme is a glycoprotein and catalyzes the transfer of the γ-glutamyl group from the glutathione (sometimes another γ-glutamyl peptide) to the transported amino acid and the subsequent transfer of the complex into the cell. Glutathione is a tripeptide - γ-glutamylcysteinylglycine, which is found in all animal tissues. The reaction proceeds as follows (see scheme A on p. 468).
The amino acid bound to the γ-glutamine residue is inside the cell. In the next reaction, γ-glutamine cleavage occurs.
Fig. 9-5.γ - Glutamyl cycle. The system consists of one membrane and five cytoplasmic enzymes. Transfer of the amino acid inside the cell is carried out in a complex with the glutamyl glutathione residue under the action of γ-glutamyltransferase. Then the amino acid is released, and the γ-glutamyl residue in several stages is converted into glutathione, which is able to add the next amino acid molecule. E 1 - γ-glutamyltransferase; E 2 - y-glutamylcyclotransferase; E 3 - peptidase; E 4 - oxoprolinase; E 5 - γ-glutamylcysteine synthetase; E 6 - glutathione synthetase.
residue under the action of the enzyme γ-glutamylcyclotransferase (see scheme B).
The cysteinylglycine dipeptide is cleaved by peptidase into 2 amino acids, cysteine and glycine. As a result of these 3 reactions, one amino acid molecule is transferred to the cell (or intracellular structure). The following 3 reactions ensure the regeneration of glutathione, due to which the cycle is repeated many times. To transport an amino acid molecule into the cell with the participation of the γ-glutamyl cycle, 3 ATP molecules are expended.
D. Violation of protein digestion and amino acid transport
Non-hydrolyzed short peptides make up a small fraction of protein digestion products. Some people have an immune reaction to a protein intake, which is obviously related to the ability to absorb
such peptides. Products completely digested protein (amino acids) are deprived of antigenic properties and do not cause immune reactions.
In newborns, the permeability of the intestinal mucosa is higher than in adults, therefore, colostrum antibodies can enter the blood (the secretion of the mammary glands secreted in the first days after birth, enriched with antibodies and antitoxins). This is aggravated by the presence of a trypsin inhibitor protein in colostrum. Proteolytic enzymes in the digestive secrets of newborns have low activity. All this contributes to the absorption in the intestine of a small amount of native proteins, sufficient to ensure an immune response. Obviously, such an increase in the absorption capacity of the intestines is the cause of sometimes observed intolerance to food proteins (eg, milk and eggs) in adults.
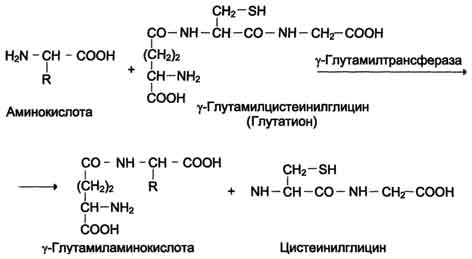
Scheme A

Scheme B
More and more evidence is being received of the hypothesis that celiac disease (non-tropical sprue)there is a violation of the cells of the intestinal mucosa, where small non-hydrolyzed peptides are absorbed. Celiac disease is characterized by hypersensitivity to gluten - a protein gluten from cereal grains used with food by humans. This protein has a toxic effect on the mucous membrane of the small intestine, which leads to its pathological changes and impaired absorption. The pathogenesis of the disease is not clear enough.
Diseases like cystinuria,disease Hartnapand some others are due to a defect in neutral amino acid carriers in the intestines and kidneys. Congenital pathology associated with a defect in the enzyme 5-oxoprolinase is described (Fig. 9-5, reaction 4). At the same time, oxoprolin is excreted in the urine. In these patients, the transport of amino acids into the tissues and their metabolism in the cells are disturbed.
Food proteins in the oral cavity are not split, as saliva does not contain hydrolytic enzymes.
Chemical cleavage of proteins begins in the stomach under the influence of proteolytic enzymes (peptide hydrolases), which cleave peptide bonds between amino acids.
These enzymes are formed by cells of the mucous membrane of the stomach, small intestine and pancreas in an inactive form. This form of enzymes prevents the self-digestion of proteins in the cells where they are synthesized, and the walls of the gastrointestinal tract (especially for those who still rave their thoughts about the fact that during fasting the stomach digests itself).
In the stomach, protein digestion occurs with the participation of the enzyme gastric juice pepsin, which is formed from inactive pepsinogen under the influence of hydrochloric acid. Pepsin exhibits maximum enzymatic activity in a strongly acidic medium at pH 1-2. In addition, under the influence of hydrochloric acid occurs swelling and partial denaturation of proteins, which leads to an increase in the surface of the contact of the enzyme with proteins. All this facilitates the process of protein breakdown in the stomach. Pepsin cleaves peptide bonds of protein molecules, resulting in the formation of high-molecular peptides and prosthetic groups.
Proteins that are not split in the small intestine, are broken down in the large intestine under the influence of peptidases, which are synthesized by the microflora located here. Enzymes of the microflora of the large intestine are able to break down many amino acids of food with the formation of various toxic substances: phenol, cresol, indole, hydrogen sulfide, mercaptans, etc. This conversion of amino acids in the large intestine is called protein decay. Toxic substances are absorbed into the bloodstream and delivered to the liver, where they are disposed of. The whole process of digesting proteins in the gastrointestinal tract takes an average of 8-12 hours after eating.
findings
A source
From the foregoing, an obvious conclusion follows: consuming carbohydrates with protein is undesirable.
There is a special sphincter in the stomach, called the pylorus, and it has a very curious function: to separate the pyloric part of the stomach from the ampulla of the duodenum and act as a regulator of the flow of acidic gastric contents into the duodenum as it is ready to receive the next chyme (food paste).
It so happened that the acidic environment of the stomach does not change, as well as the alkaline environment of intestinal juice. Therefore, by making a vinaigrette, you doom yourself to one of the following outcomes:
1) When the pylorus of the stomach does not let food into the intestine until the protein is digested, this delays the entry of glucose into the blood, and, much worse, you can ferment carbohydrates. It threatens to break down carbohydrates into carbon dioxide, acetic acid, and alcohol - substances that are not suitable for absorption.
2) When most of the food eaten is made up of carbohydrates, and carefully mixing them with protein, you drag the whole thing into the intestines ahead of time, which will result in further rotting of the protein in the large intestine. The stomach does not know the mechanism to separate these thoroughly mixed substances and place them in different parts of their cavity.
The fact that protein decay is constantly observed in the large intestine of a “civilized” person does not mean that such a phenomenon can be considered normal. And rotting is smelly feces, difficult stools, bloated abdomen and gas.
To summarize, do as follows:
· First of all, eat all carbohydrates, chewing very carefully, because digestion will begin in the oral cavity. Because of the acidic environment in the stomach, carbohydrates will not linger there for a long time, by the time you are on the protein side, most carbohydrates will already fall into the intestines (for greater reliability, it is better to pause for 15-20 minutes).
· Fats, for nuts, butter products, etc., are best used with carbohydrates, because alkaline medium is needed and because lipids slow down gastric secretion, thereby inhibiting digestion. In the case when the fat cannot be separated from the protein, for example, the oil used in the preparation, it is possible to stop this ailment with raw green vegetables.
· Proteins, respectively, are the last number.
Dividing and eating food in this sequence, you kill three birds with one stone:
1. occupy the intestines with organic substances that perform an energy function;
2. occupy the stomach main carriers of life;
3. and what is important, in this way you can stupidly eat more (relevant to periodic starvation).
To take the word of a textbook of physiology, a textbook of biochemistry, and even more so I don’t call on anyone personally, just try and form your own opinion.